The Biology of Behavior
Northwestern scientists probe the molecular underpinnings of human behavior and neurological disorders
By Olivia Dimmer
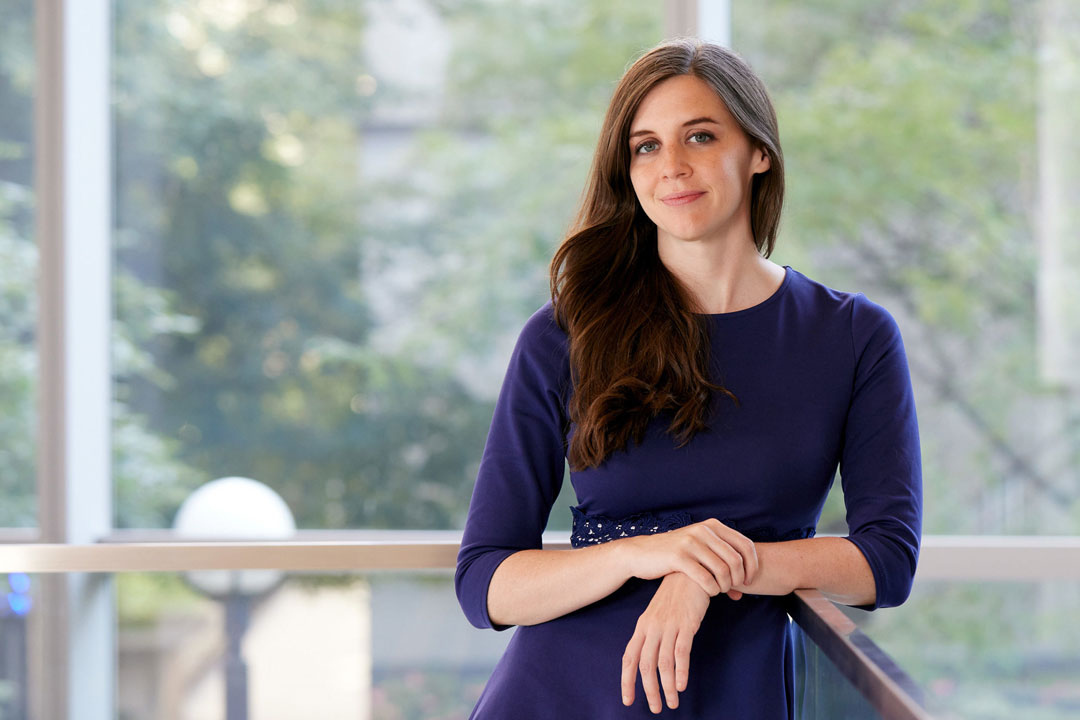
Understanding how the activity of the billions of neurons in our brain translates to human behavior – from micro decisions to compulsive habits – has been a huge, decades-long investigation. Yet many of these molecular mechanisms remain elusive even to the scientists and doctors studying them.
To accelerate discovery at the intersection of neuroscience and behavior, Feinberg recently launched the Center for Psychiatric Neuroscience, a collaborative hub created to unite interdisciplinary scientists to understand neural mechanisms underlying mental illness and develop novel therapeutics. Sachin Patel, MD, PhD, the chair and Lizzie Gilman Professor of Psychiatry and Behavioral Sciences, will serve as director of the Center for Psychiatric Neuroscience.
Cultivating a Department of Psychiatry integrated with robust translational neuroscience research is extremely important for the future of our field.
Sachin Patel, MD, PhD
“Cultivating a Department of Psychiatry integrated with robust translational neuroscience research is extremely important for the future of our field,” Patel says. “This is a unique opportunity to synergize with the existing neuroscience infrastructure including the Departments of Neuroscience, Pharmacology, Neurobiology and other areas to establish a center that’s really at the intersection between mental health and neuroscience.”
In addition to serving as a platform for recruiting new faculty to Feinberg, the center will be a focal point for current Northwestern faculty with an interest in using neuroscience approaches to better understand pathophysiological mechanisms of mental illness.
The new center builds on recent high-impact research at Northwestern Medicine, which highlights the complex, intertwined nature of brain circuitry and behavior patterns.
BREAKTHROUGHS IN AUTISM
An estimated 1 in 36 children in the U.S. live with autism, according to the Centers for Disease Control and Prevention, and roughly 1 percent of the world’s population has autism spectrum disorder.
In the laboratory of Peter Penzes, PhD, director of the Center for Autism and Neurodevelopment and the Ruth and Evelyn Dunbar Professor of Psychiatry and Behavioral Sciences, multiple new studies have uncovered the complex relationship between genetics and the molecular mechanisms underlying behavior in autism disorders.
Recently, Penzes and his team have uncovered how social deficits and seizures in a subtype of autism are caused by overexcited brain circuits. In a study published in Nature Communications, they found that a subtype of autism called duplication syndrome was linked to increased levels of the gene PRRT2 in mice, causing seizures and abnormal social behaviors.
When levels of PRRT2 were reduced in the duplicated region, brain activity in mice returned to normal, expected social behavior was restored, and seizures decreased.
Because the gene PRRT2 regulates how neurons talk to each other, inhibiting synapses or connection points between neurons could help treat both seizures and autism symptoms in this syndrome, says lead author Marc Forrest, PhD, research assistant professor of Neuroscience and lead author of the study. This approach could also be used more broadly in other types of neurodevelopmental disorders with brain over-activation, which has been shown in other subtypes of autism.
Our work now shows that we can focus our efforts on targeting the PRRT2 pathway for novel therapies, and these could potentially cure core symptoms of 16p11.2 duplication syndrome.
Marc Forrest, PhD
“Our work now shows that we can focus our efforts on targeting the PRRT2 pathway for novel therapies, and these could potentially cure core symptoms of 16p11.2 duplication syndrome,” Forrest says. “If we learn how the 16p11.2 duplication causes illness, maybe we can also learn more about what causes autism in general and create better treatments.”
Penzes and his collaborators have also identified new biomarkers within patients’ cerebrospinal fluid (CSF) for a type of autism, according to a study published in the journal Neuron.
This biomarker’s presence helps establish a link between autism and epilepsy, conditions which often co-occur but whose conjunctive mechanisms remain unclear. For example, people with autism with mutations in the gene CNTNAP2 also have epilepsy. Normally, this gene creates a cellular adhesion protein which helps neurons connect to one another, but the basal ganglia that are responsible for controlling movement and reward-seeking behaviors, is connected to the brain’s cerebral cortex via corticostriatal circuits. Prior research suggested these circuits control the expression of compulsive behaviors commonly observed in obsessive-compulsive disorder (OCD) and substance use disorders.
In the Neuron study, Penzes and his collaborators analyzed cerebrospinal fluid from both autistic patients and a control group, finding that autistic patients had fewer molecules of CNTNAP2 floating freely in the CSF. When CNTNAP2 is freely floating, it functions more like a hormone than a cellular glue, Penzes said, binding to neurons and reducing excitatory neurotransmissions. Therefore, CNTNAP2 may play a secondary role as a regulator of excitatory activity.
The team also studied another genetic mutation, this time in the gene SHANK3, whichled to the development of a new therapy for Phelan-McDermid syndrome, a subtype of autism spectrum disorder (ASD).
Phelan-McDermid syndrome is known to be caused by a specific genetic mutation in SHANK3, a well-known ASD candidate gene. Penzes’ team developed a derivative of an insulin-like growth factor-binding protein, IGFBP2, which is found in structures of the brain affected by ASD and has been shown to improve neuroplasticity and cognitive functions.
In a study published in Molecular Psychiatry, the investigators administered the IGFBP2-derived peptide, called JB2, to mice with SHANK3 mutations. Through advanced brain imaging, they found that the drug improved neuroplasticity, behavior impairments, and cellular processes in the mice’s brains.
These changes were directly correlated with improvements in the mice’s learning and memory skills, motor function, and communication through ultrasonic vocalizations. While the drug is still in early stages, Penzes said, physicians could theoretically routinely administering the drug into patients’ bloodstreams.
BRAIN CIRCUITRY AND SEX DIFFERENCES IN DECISION-MAKING
Humans make thousands of decisions each day – from what to eat to whom to marry. Research has shown that decision-making behaviors vary by sex, but the exact neural mechanisms that mediate these differences have remained poorly understood.
Investigators led by Julia Cox, PhD, research assistant professor of Neuroscience, have shed new light on the neural mechanisms that influence how sex and past experiences inform decision making, according to findings published in Nature Neuroscience.
In the study, investigators gave mice the choice of two levers to press: one yielded a reward, the other did not. Through trial and error, the mice ultimately used their past experiences to figure out which lever was more likely to give them a reward. Once the mice were trained in this task, the investigators performed two different experiments. In the first, the team implanted optic fibers into the mice brains, allowing investigators to decrease neuronal activity with a light-activated protein and determine how these changes in neuronal activity influenced the mice’s behavior.
In the second experiment, the team used miniaturized high-resolution microscopic lenses while the mice performed tasks, which allowed investigators to use advanced imaging techniques and analyze when subsets of neurons were activated during the tasks.
While all the mice exhibited similar behavior, Cox and her collaborators noticed one stark difference: Female mice were more strongly influenced by “action value,” or how likely one is to receive a reward for an action based on past experiences, than male mice.
“The initial goal of this experiment was not to find a sex difference, so we were pretty surprised by that,” Cox said.
Cox’s team also found that inhibiting activity in neurons that project from the anterior cingulate cortex to the dorsomedial striatum — both areas of the brain essential for decision-making — disrupted the relationship between value and motivation in the female mice.
After replicating these experiments with human participants, the investigators discovered that female participants were also influenced by the action value of previous tasks were slower to reengage in the task based on whether or not the previous task yielded a positive outcome.
“In that regard, there are similarities,” Cox said. “I think that more broadly, when studying sex differences in laboratory animals, even if the behavioral differences don’t always end up translating to humans, it’s possible that some of the differences in the neural circuits still are consistent across species.”
DOPAMINE’S LINK TO HABIT FORMATION
Compulsive behavior is a hallmark of both addiction and obsessive compulsive disorder, which can cause a person to have urges that trigger intensely distressing feelings or perform repetitive behaviors that are unwanted and can interfere with daily life.
Northwestern investigators have discovered that dopamine signaling in the brain’s dorsomedial striatum promotes the development of compulsive behaviors in animal models, according to findings published in Current Biology.
The striatum, a cluster of neurons within the basal ganglia that are responsible for controlling movement and reward-seeking behaviors, is connected to the brain’s cerebral cortex via corticostriatal circuits. Prior research suggested these circuits control the expression of compulsive behaviors commonly observed in obsessive-compulsive disorder (OCD) and substance use disorders.
While corticostriatal circuits were thought be involved, the precise mechanisms that cause compulsive behaviors to emerge have remained unclear.
Talia Lerner, PhD, assistant professor of Neuroscience and senior author of the study, hypothesized that dopamine activity, which regulates the plasticity of corticostriatal circuits, played a crucial role in this process.
In the study, investigators studied dopamine activity in two regions of the brain’s striatum: the dorsomedial striatum and the dorsolateral striatum, both involved in different types of reward learning. The dorsomedial striatum is involved with goal-oriented learning behaviors, while the dorsolateral stratum is involved with habitual behaviors.
Although it was previously thought that habit played a role in compulsion, the team was surprised to find that dopamine signaling activity was actually upregulated in the mice’s dorsomedial striatum, where it predicted the development of compulsive reward-seeking behavior.
The investigators then used excitatory and inhibitory optogenetics — which uses light to change the activity of neurons — to manipulate the activity of dopamine neurons in the dorsomedial striatum. They found that stimulating dopamine signaling in the dorsomedial striatum increased compulsive reward seeking, while inhibiting dopamine signaling in the dorsomedial striatum decreased compulsive reward seeking, confirming that the associations they had observed were causal.
The findings can help scientists understand the root causes behind compulsive behaviors, which may help physicians better determine which management strategies will work best for individual patients.
AGGRESSION AS A DIAL, NOT A SWITCH
Aggression between members of a species is as old as time, but involves careful calculations, Ann Kennedy, PhD, assistant professor of Neuroscience, wrote in an essay published in Science.
Fighting can leave an animal injured, so it’s safer to start out with aggressive posturing, and only escalate to outright fighting if an opponent doesn’t back down. Scientists therefore expect aggressive arousal to build over time and to persist even after a fight is over, but how this is accomplished in the brain is still relatively unknown.
To better understand this process, Kennedy and her colleagues used head-mounted miniaturized microendoscopes to characterize the activity of neurons in the ventrolateral portion of the ventromedial hypothalamus as mice freely interacted.
The investigators observed the activity in these cells was only weakly correlated with the mice’s actions. But by fitting the data with a dynamical systems model, they uncovered a subset of neurons showing gradual ramping of their activity, including persistent firing after a fight ended.
When the activity of these neurons were weak, mice investigated or ignored one another, but as the activity grew, so did aggressive posturing, such as dominance mounting. When activity in the neurons peaked, mice began to attack. Furthermore, mice that never fought showed only weak ramping activity of these neurons, whereas intense fighters showed strong and long-lasting ramping.
Kennedy’s research suggests the signals present in these neurons reflect a level of aggressive motivation and argues that the scalable and persistent activity within the ventrolateral portion of the ventromedial hypothalamus is a mechanism for setting an animal’s motivational state.
“We had this question: ‘What makes us behave differently when we’re angry or hungry? How does the brain encode these internal motivational states?’” Kennedy says. “What we found was if you look at activity of these hypothalamic neurons, you can pull out this signal that gradually ramps up over the course of an aggressive counter, and is correlated with animals’ overall level of aggression. It’s not a decision to attack, but it’s like this scalable knob of how ‘angry’ the mouse is, that gets cranked up over the course of an interaction.”